Snapshot of - C3IAM
Archive of C3IAM, version: 2.0
Reference card - C3IAM
The reference card is a clearly defined description of model features. The numerous options have been organized into a limited amount of default and model specific (non default) options. In addition some features are described by a short clarifying text.
Legend:
- not implemented
- implemented
- implemented (not default option)
About
Name and version
C3IAM 2.0
Model link
Institution
Center for Energy and Environmental Policy Research, Beijing Institute of Technology (CEEP-BIT), China, http://ceep.bit.edu.cn/english/.
Documentation
C3IAM documentation consists of a referencecard and detailed model documentation
Process state
published
Model scope and methods
Model documentation: Model scope and methods - C3IAM
Model type
- Integrated assessment model
- Energy system model
- CGE
- CBA-integrated assessment model
Geographical scope
- Global
- Regional
Objective
assessing the impacts of climate policies in the future development of a complex and dynamic system
Solution concept
- Partial equilibrium (price elastic demand)
- Partial equilibrium (fixed demand)
- General equilibrium (closed economy)
Solution horizon
- Recursive dynamic (myopic)
- Intertemporal optimization (foresight)
Solution method
- Simulation
- Optimization
Temporal dimension
Base year:2011, time steps:5, horizon: 2011-2100
Spatial dimension
Number of regions:12
- USA
- China
- Japan
- Russian Federation
- India
- Other Branches of Umbrella Group
- European Union
- Other West European Developed Countries
- Eastern European CIS excluding Russian Federation
- Asia excluding China,India and Japan
- Middle East and Africa
- Latin America
Time discounting type
- Discount rate exogenous
- Discount rate endogenous
Policies
- Emission tax
- Emission pricing
- Cap and trade
- Fuel taxes
- Fuel subsidies
- Feed-in-tariff
- Portfolio standard
- Capacity targets
- Emission standards
- Energy efficiency standards
- Agricultural producer subsidies
- Agricultural consumer subsidies
- Land protection
- Pricing carbon stocks
Socio-economic drivers
Model documentation: Socio-economic drivers - C3IAM
Population
- Yes (exogenous)
- Yes (endogenous)
Population age structure
- Yes (exogenous)
- Yes (endogenous)
Education level
- Yes (exogenous)
- Yes (endogenous)
Urbanization rate
- Yes (exogenous)
- Yes (endogenous)
GDP
- Yes (exogenous)
- Yes (endogenous)
Income distribution
- Yes (exogenous)
- Yes (endogenous)
Employment rate
- Yes (exogenous)
- Yes (endogenous)
Labor productivity
- Yes (exogenous)
- Yes (endogenous)
Total factor productivity
- Yes (exogenous)
- Yes (endogenous)
Autonomous energy efficiency improvements
- Yes (exogenous)
- Yes (endogenous)
Macro-economy
Model documentation: Macro-economy - C3IAM
Economic sector
Industry
- Yes (physical)
- Yes (economic)
- Yes (physical & economic)
Energy
- Yes (physical)
- Yes (economic)
- Yes (physical & economic)
Transportation
- Yes (physical)
- Yes (economic)
- Yes (physical & economic)
Residential and commercial
- Yes (physical)
- Yes (economic)
- Yes (physical & economic)
Agriculture
- Yes (physical)
- Yes (economic)
- Yes (physical & economic)
Forestry
- Yes (physical)
- Yes (economic)
- Yes (physical & economic)
Macro-economy
Trade
- Coal
- Oil
- Gas
- Uranium
- Electricity
- Bioenergy crops
- Food crops
- Capital
- Emissions permits
- Non-energy goods
Cost measures
- GDP loss
- Welfare loss
- Consumption loss
- Area under MAC
- Energy system cost mark-up
Categorization by group
- Income
- Urban - rural
- Technology adoption
- Age
- Gender
- Education level
- Household size
Institutional and political factors
- Early retirement of capital allowed
- Interest rates differentiated by country/region
- Regional risk factors included
- Technology costs differentiated by country/region
- Technological change differentiated by country/region
- Behavioural change differentiated by country/region
- Constraints on cross country financial transfers
Resource use
Coal
- Yes (fixed)
- Yes (supply curve)
- Yes (process model)
Conventional Oil
- Yes (fixed)
- Yes (supply curve)
- Yes (process model)
Unconventional Oil
- Yes (fixed)
- Yes (supply curve)
- Yes (process model)
Conventional Gas
- Yes (fixed)
- Yes (supply curve)
- Yes (process model)
Unconventional Gas
- Yes (fixed)
- Yes (supply curve)
- Yes (process model)
Uranium
- Yes (fixed)
- Yes (supply curve)
- Yes (process model)
Bioenergy
- Yes (fixed)
- Yes (supply curve)
- Yes (process model)
Water
- Yes (fixed)
- Yes (supply curve)
- Yes (process model)
Raw Materials
- Yes (fixed)
- Yes (supply curve)
- Yes (process model)
Land
- Yes (fixed)
- Yes (supply curve)
- Yes (process model)
Technological change
Energy conversion technologies
- No technological change
- Exogenous technological change
- Endogenous technological change
Energy End-use
- No technological change
- Exogenous technological change
- Endogenous technological change
Material Use
- No technological change
- Exogenous technological change
- Endogenous technological change
Agriculture (tc)
- No technological change
- Exogenous technological change
- Endogenous technological change
Energy
Model documentation: Energy - C3IAM
Energy technology substitution
Energy technology choice
- No discrete technology choices
- Logit choice model
- Production function
- Linear choice (lowest cost)
- Lowest cost with adjustment penalties
Energy technology substitutability
- Mostly high substitutability
- Mostly low substitutability
- Mixed high and low substitutability
Energy technology deployment
- Expansion and decline constraints
- System integration constraints
Energy
Electricity technologies
- Coal w/o CCS
- Coal w/ CCS
- Gas w/o CCS
- Gas w/ CCS
- Oil w/o CCS
- Oil w/ CCS
- Bioenergy w/o CCS
- Bioenergy w/ CCS
- Geothermal power
- Nuclear power
- Solar power
- Solar power-central PV
- Solar power-distributed PV
- Solar power-CSP
- Wind power
- Wind power-onshore
- Wind power-offshore
- Hydroelectric power
- Ocean power
Hydrogen production
- Coal to hydrogen w/o CCS
- Coal to hydrogen w/ CCS
- Natural gas to hydrogen w/o CCS
- Natural gas to hydrogen w/ CCS
- Oil to hydrogen w/o CCS
- Oil to hydrogen w/ CCS
- Biomass to hydrogen w/o CCS
- Biomass to hydrogen w/ CCS
- Nuclear thermochemical hydrogen
- Solar thermochemical hydrogen
- Electrolysis
Refined liquids
- Coal to liquids w/o CCS
- Coal to liquids w/ CCS
- Gas to liquids w/o CCS
- Gas to liquids w/ CCS
- Bioliquids w/o CCS
- Bioliquids w/ CCS
- Oil refining
Refined gases
- Coal to gas w/o CCS
- Coal to gas w/ CCS
- Oil to gas w/o CCS
- Oil to gas w/ CCS
- Biomass to gas w/o CCS
- Biomass to gas w/ CCS
Heat generation
- Coal heat
- Natural gas heat
- Oil heat
- Biomass heat
- Geothermal heat
- Solarthermal heat
- CHP (coupled heat and power)
Grid Infra Structure
Electricity
- Yes (aggregate)
- Yes (spatially explicit)
Gas
- Yes (aggregate)
- Yes (spatially explicit)
Heat
- Yes (aggregate)
- Yes (spatially explicit)
CO2
- Yes (aggregate)
- Yes (spatially explicit)
Hydrogen
- Yes (aggregate)
- Yes (spatially explicit)
Energy end-use technologies
Passenger transportation
- Passenger trains
- Buses
- Light Duty Vehicles (LDVs)
- Electric LDVs
- Hydrogen LDVs
- Hybrid LDVs
- Gasoline LDVs
- Diesel LDVs
- Passenger aircrafts
Freight transportation
- Freight trains
- Heavy duty vehicles
- Freight aircrafts
- Freight ships
Industry
- Steel production
- Aluminium production
- Cement production
- Petrochemical production
- Paper production
- Plastics production
- Pulp production
Residential and commercial
- Space heating
- Space cooling
- Cooking
- Refrigeration
- Washing
- Lighting
Land-use
Model documentation: Land-use - C3IAM
Land cover
- Cropland
- Cropland irrigated
- Cropland food crops
- Cropland feed crops
- Cropland energy crops
- Forest
- Managed forest
- Natural forest
- Pasture
- Shrubland
- Built-up area
Agriculture and forestry demands
- Agriculture food
- Agriculture food crops
- Agriculture food livestock
- Agriculture feed
- Agriculture feed crops
- Agriculture feed livestock
- Agriculture non-food
- Agriculture non-food crops
- Agriculture non-food livestock
- Agriculture bioenergy
- Agriculture residues
- Forest industrial roundwood
- Forest fuelwood
- Forest residues
Agricultural commodities
- Wheat
- Rice
- Other coarse grains
- Oilseeds
- Sugar crops
- Ruminant meat
- Non-ruminant meat and eggs
- Dairy products
Emission, climate and impacts
Model documentation: Emissions - C3IAM, Climate - C3IAM, Non-climate sustainability dimension - C3IAM
Greenhouse gases
- CO2 fossil fuels
- CO2 cement
- CO2 land use
- CH4 energy
- CH4 land use
- CH4 other
- N2O energy
- N2O land use
- N2O other
- CFCs
- HFCs
- SF6
- PFCs
Pollutants
- CO energy
- CO land use
- CO other
- NOx energy
- NOx land use
- NOx other
- VOC energy
- VOC land use
- VOC other
- SO2 energy
- SO2 land use
- SO2 other
- BC energy
- BC land use
- BC other
- OC energy
- OC land use
- OC other
- NH3 energy
- NH3 land use
- NH3 other
Climate indicators
- Concentration: CO2
- Concentration: CH4
- Concentration: N2O
- Concentration: Kyoto gases
- Radiative forcing: CO2
- Radiative forcing: CH4
- Radiative forcing: N2O
- Radiative forcing: F-gases
- Radiative forcing: Kyoto gases
- Radiative forcing: aerosols
- Radiative forcing: land albedo
- Radiative forcing: AN3A
- Radiative forcing: total
- Temperature change
- Sea level rise
- Ocean acidification
Carbon dioxide removal
- Bioenergy with CCS
- Reforestation
- Afforestation
- Soil carbon enhancement
- Direct air capture
- Enhanced weathering
Climate change impacts
- Agriculture
- Energy supply
- Energy demand
- Economic output
- Built capital
- Inequality
Co-Linkages
- Energy security: Fossil fuel imports & exports (region)
- Energy access: Household energy consumption
- Air pollution & health: Source-based aerosol emissions
- Air pollution & health: Health impacts of air Pollution
- Food access
- Water availability
- Biodiversity
Model Documentation - C3IAM
The C3IAM is an integrated assessment model framework of interacting social economic system and earth system, which dynamically captures the large-scale and long-term optimal economic growth and climate change mitigation & adaptation. C3IAM consists of a combination of seven different models or modules, including the Global Energy and Environmental Policy Analysis model (C3IAM/GEEPA), the Global Multi-regional Economic Optimum Growth model (C3IAM/EcOp), the China’s Multi-Regional Energy and Environmental Policy Analysis model (C3IAM/MR.CEEPA), the National Energy Technology model (C3IAM/NET), the Climate System model (C3IAM/Climate), the Ecological Land Use model (C3IAM/EcoLa) and the Climate Change Loss model (C3IAM/Loss), which complement each other and are specialized in different areas. The seven models are interlinked within the integrated framework.
1) Model scope and methods - C3IAM
C3IAM consists of various analytical models developed to analyze policy issues within a specific set of sectors as shown in Figure 1[1]. These models are interlinked to provide an integrated system for assessing the impact of climate change. C3IAM considers factors such as global multi-regional, multi-sectoral economic development, greenhouse gas emissions, emission reduction costs, climate change losses module, etc. It can not only depict the social economic system in detail, but also realize a long-term balanced growth path.
To apply C3IAM, all model settings are adjusted so that the model reproduces the state-of-the-world in 2011 and cover the period 2011–2100.
C3IAM pays more attention to clarify the comprehensive impacts of climate change and it has a better performance in the following various aspects:
1. More in-depth depiction of China: to refine the emissions pathway from the perspective of regional and sectoral, the Multi-Regional CGE Model (C3IAM/MR.CEEPA) that covers 31 provinces and the multi-sector technology model (C3IAM/NET) that covers eight energy-intensive industries are developed;
2. Extension of economic model: to capture the long-term optimal economic growth and climate change mitigation dynamically, C3IAM integrates the global CGE model (C3IAM/GEEPA) and the global economic optimum growth model (C3IAM/EcOp);
3. Realizing the hard link between the earth and socioeconomic systems: the economic models are integrated with earth system model, and the two-way feedbacks could be achieved.
<figure id="fig:The general structure of C3IA">
</figure>
References
- ↑ Yi-Ming Wei, Rong Han, Qiao-Mei Liang, Bi-Ying Yu, Yun-Fei Yao, Mei-Mei Xue, Kun Zhang, Li-Jing Liu, Juan Peng, Pu Yang, Zhi-Fu Mi, Yun-Fei Du, Ce Wang, Jun-Jie Chang, Qian-Ru Yang, Zili Yang, Xueli Shi, Wei Xie, Changyi Liu, Zhongyu Ma, Jinxiao Tan, Weizheng Wang, Bao-Jun Tang, Yun-Fei Cao, Mingquan Wang, Jin-Wei Wang, Jia-Ning Kang, Ke Wang, Hua Liao, 2018. An integrated assessment of INDCs under Shared Socioeconomic Pathways: an implementation of C3IAM. Natural Hazards 92, 585-618.
1.1) Model concept, solver and details - C3IAM
C3IAM belongs to a class of integrated assessment model. In C3IAM, there are multiple independent but interactive modules, including three socio-economic modules (GEEPA, MR.CEEPA and EcOp), one technology module for China (NET), one land-use module (EcoLa), one climate module (Climate) and one loss module (Loss). Thus C3IAM integrates the global CGE, economic optimum growth, revised earth system, land use and impact models, dynamically captures the long-term optimal economic growth and climate change mitigation and adaptation. C3IAM is a flexible framework that can be applied to a variety of economy-climate-energy-environment issues. The trade of goods and services is also considered.
In C3IAM, GEEPA and MR.CEEPA are implemented for GAMS/MCP (Mixed Complementarity Problem), and PATH is used as a solver; EcOp is implemented for GAMS/NLP (Non-Linear Programming, and the solver is MINOS; NET is implemented for GAMS/LP (Linear Programming) and the solver is CPLEX; the parameters in Climate module are calculated with R and EcoLa is run by MATLAB. Generally, the C3IAM is a dynamic model using a 5-year time step.
1.3) Temporal dimension - C3IAM
C3IAM is a dynamic inter-temporal model with the time horizon from 2011 to 2100 focusing on long-term trends to capture the comprehensive impacts of climate change. The spacing of time steps is flexible. In the default case, there are five-year steps (2011, 2015, 2020, 2025, 2030, 2035, 2040, 2045, 2050, 2055, 2060, 2065, 2070, 2075, 2080, 2085, 2090, 2095, and 2100).
1.4) Spatial dimension - C3IAM
C3IAM framework has global coverage and divides the world into 12 regions, which are USA, China, Japan, Russian Federation, India, Other Branches of Umbrella Group, European Union, Other West European Developed Countries, Eastern European CIS excluding Russian Federation, Asia excluding China, India and Japan, Middle East and Africa and Latin America (See Figure 1 and Table 1 below). <figure id="Figure 1. The classification of regions in C3IAM">
</figure>
Region | Involved members |
USA | United States of America |
CHN | China |
JPN | Japan |
RUS | Russian Federation |
IDN | India |
OBU
(Other Branches of Umbrella Group) |
Canada, Australia, New Zealand |
EU
(European Union) |
Austria, Belgium, Denmark, Finland, France, Germany, Greece, Ireland, Italy, Luxembourg, Netherlands, Portugal, Spain, Sweden, United Kingdom, Cyprus, Czech Republic, Estonia, Hungary, Malta, Poland, Slovakia, Slovenia, Bulgaria, Latvia, Lithuania, Romania, Croatia |
OWE
(Other West European Developed Countries) |
Albania, Montenegro, Serbia, The former Yugoslav Republic of Macedonia, Turkey, Bosnia and Herzegovina, Guam, Iceland, Liechtnstein, Norway, Puerto Rico, Switzerland, |
EES
(Eastern European CIS excluding Russian Federation) |
Armenia, Azerbaijan, Belarus, Georgia, Kazakhstan, Kyrgyzstan, Republic of Moldova, Tajikistan, Turkmenistan, Ukraine, Uzbekistan |
ASIA
(Asia excluding China, India, Japan) |
Afghanistan, Bangladesh, Bhutan, Brunei Darussalam, Cambodia, Democratic People's Republic of Korea, Fiji, French Polynesia, Indonesia, Lao People's Democratic Republic, Malaysia, Maldives, Micronesia (Fed. States of), Mongolia, Myanmar, Nepal, New Caledonia, Pakistan, Papua New Guinea, Philippines, Republic of Korea, Samoa, Singapore, Solomon Islands, Sri Lanka, Taiwan (Province of China), Thailand, Timor-Leste, Vanuatu, Viet Nam |
MAF
(Middle East and Africa) |
Algeria, Angola, Bahrain, Benin, Botswana, Burkina Faso, Burundi, Cameroon, Cape Verde, Central African Republic, Chad, Comoros, Congo, Côte d`Ivoire, Democratic Republic of the Congo, Djibouti, Egypt, Equatorial Guinea, Eritrea, Ethiopia, Gabon, Gambia, Ghana, Guinea, Guinea-Bissau, Iran (Islamic Republic of), Iraq, Israel, Jordan, Kenya, Kuwait, Lebanon, Lesotho, Liberia, Libyan Arab Jamahiriya, Madagascar, Malawi, Mali, Mauritania, Mauritius, Mayotte, Morocco, Mozambique, Namibia, Niger, Nigeria, Occupied Palestinian Territory, Oman, Qatar, Rwanda, Réunion, Saudi Arabia, Senegal, Sierra Leone, Somalia, South Africa, South Sudan, Sudan, Swaziland, Syrian Arab Republic, Togo, Tunisia, Uganda, United Arab Emirates, United Republic of Tanzania, Western Sahara, Yemen, Zambia, Zimbabwe |
LAM
(Latin America) |
Argentina, Aruba, Bahamas, Barbados, Belize, Bolivia (Plurinational State of), Brazil, Chile, Colombia, Costa Rica, Cuba, Dominican Republic, Ecuador, El Salvador, French Guiana, Grenada, Guadeloupe, Guatemala, Guyana, Haiti, Honduras, Jamaica, Martinique, Mexico, Nicaragua, Panama, Paraguay, Peru, Suriname, Trinidad and Tobago, United States Virgin Islands, Uruguay, Venezuela (Bolivarian Republic of) |
1.5) Policy - C3IAM
C3IAM can assess a number of different economy-, energy-, environment- and climate-related policies, depending on the scenario setup and the research topic. Key areas where policy responses can be introduced into the model are:
- Carbon emission tax
- Carbon emission quotas and trading permits
- Share of technology in power, iron and steel cement, transport, buildings and chemical industries
- Low/zero/negative carbon subsidies
- Pollution/Energy taxes
- Trade policies (e.g. import tariff change
- Land use and agriculture policies
- Cooperation mechanisms on climate mitigation
- Other policies (e.g. income tax change, subsidy change and so on )
2) Socio-economic drivers - C3IAM
Socio-economic drivers are typically informed by a scenario narrative that describes in qualitative terms the overall logic behind the scenarios [1]. In C3IAM, the Shared Socio-economic Pathways (SSPs, see O’Neill et al. (2014) [1]) provide the overall scenario logic with which the main socio-economic drivers, i.e. population and GDP, have been quantified. SSPs have enriched the social economic background with a range of socioeconomic drivers’ projections (e.g., GDP, population, and urbanization rate). Previous studies such as O’Neill et al. (2014) [1] have presented narrative descriptions, which are a set of five qualitative descriptions of future changes in demographics, human development, economy and lifestyle, policies and institutions, technology, as well as environment and natural resources.
C3IAM’s default population projections are based on those of the International Institute for Applied Systems Analysis (IIASA) and the National Center for Atmospheric Research (NCAR). The GDP scenarios are projected by the Organization for Economic Cooperation and Development (OECD).These projections are available for all five different SSP scenarios. For default scenarios, C3IAM uses SSP2 data as they represent a middle-of-the road scenario.
References
2.1) Population - C3IAM
Demographic development affects strongly the implications for the anticipated mitigation and adaptation challenges. Population and labor forces are exogenous parameters in C3IAM and are based on the Shared Socio-economic Pathways (SSPs) at the country level SSP database made available by IIASA. The exogenous labor input affects the dynamics of other macroeconomic production factors (capital, energy) since the model seeks an optimal allocation of production factors.
2.2) Economic activity - C3IAM
In addition to population, economic development has a strong impact on the challenges to mitigation and adaptation. The future macro-economic assumption also causes changes in the supply and demand of goods. In C3IAM, there are two ways of treatment of macroeconomic assumption differentiating between baseline and mitigation scenarios. In baseline scenario, GDP is assumed as exogenous, while total factor productivity (TFP) is assumed as endogenous. Therefore, the GDP assumption is used to calibrate the TFP. In mitigation scenario, we set the TFP values which is calibrated in baseline scenarios as exogenous.
3) Macro-economy - C3IAM
C3IAM framework includes three macro-economy modules: the Global Multi-regional Economic Optimum Growth model (C3IAM/EcOp), the Global Energy and Environmental Policy Analysis model (C3IAM/GEEPA) and the Multi-Regional China Energy and Environmental Policy Analysis model (C3IAM/MR.CEEPA).
The C3IAM/EcOp is established based on the theory of optimal economic growth and consists of two modules (economic and climate modules) (as shown in Figure 1). The economic module describes the optimal economic growth path and investment decisions under the balance of long-term emission reduction costs and climate losses[1].
While the climate module, which is a simplified model by upscaling C3IAM/Climate, presents the greenhouse gas concentration growth, radiative forcing and temperature change thereafter. The mitigation, adaptation and loss module is simplified based on upscaling C3IAM/Loss. To maximize global welfare, the model optimizes regional consumption and investment. Therefore, national optimal mitigation and adaptation decisions could be provided.
The assumptions, model structure and mathematical formulae between C3IAM/GEEPA and C3IAM/MR.CEEPA are similar. Both are composed of five basic modules, i.e., production, income, expenditure, investment and foreign trade module. The production module describes the production structure in different regions, in which the input in each sector assumes to follow a nested constant elasticity of substitute (CES) function. The household income mainly comes from labor income and capital returns. We assume that after paying household income tax, households spend disposable income on saving and on the consumption of various goods. Household saving is obtained by multiplying household disposable income with saving rate. The consumption, production and trade of goods and services are fundamentally determined by market prices. Capital and labor allocation is determined by wages and return of capital. The basic frameworks for C3IAM/GEEPA and C3IAM/MR.CEEPA are shown in Figure 2 and Figure 3, respectively.
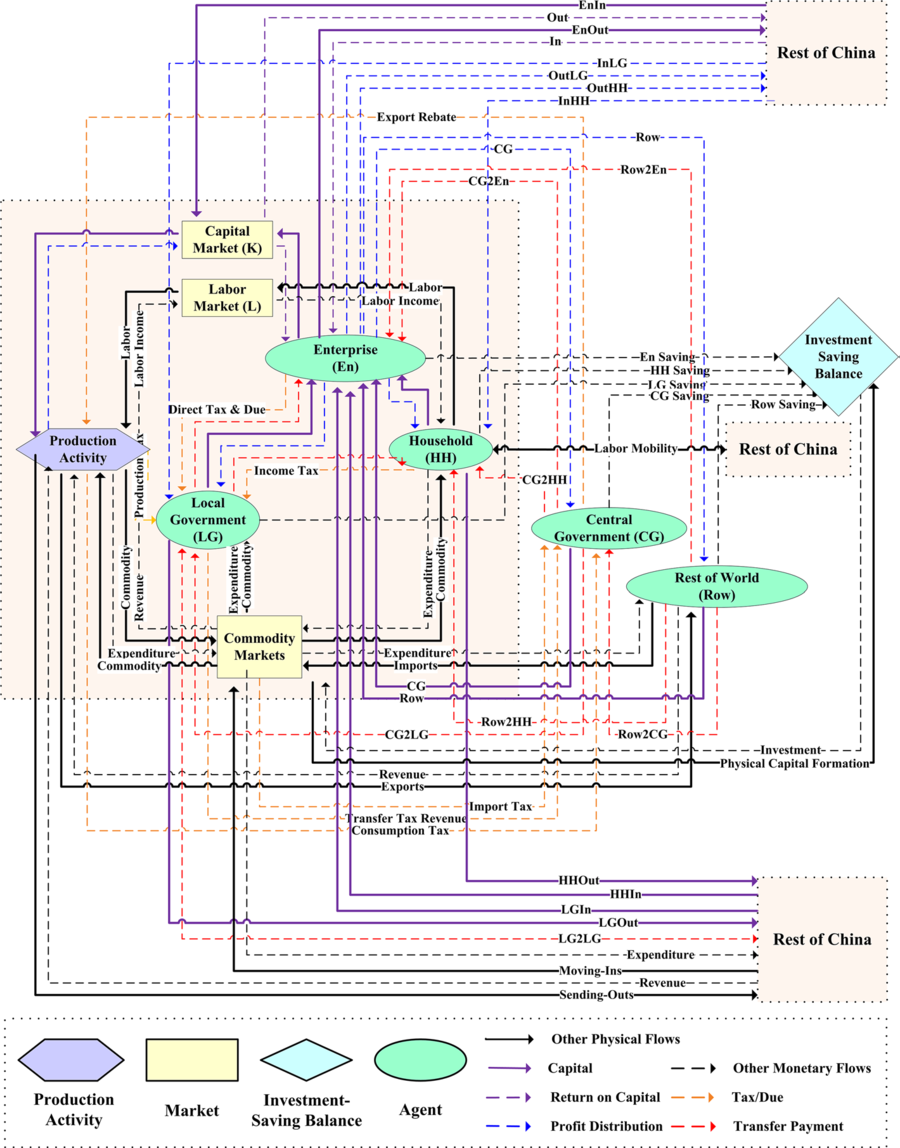
References
- ↑ Yi-Ming Wei, Rong Han, Ce Wang, Biying Yu, Qiao-Mei Liang, Xiao-Chen Yuan, Junjie Chang, Qingyu Zhao, Hua Liao, Baojun Tang, Jinyue Yan, Lijing Cheng, Zili Yang, 2020. Self-preservation strategy for approaching global warming targets in the post-Paris Agreement era. Nature Communications 11, 1624.
- ↑ Kun Zhang, Qiao-Mei Liang, Li-Jing Liu, Mei-Mei Xue, Bi-Ying Yu, Ce Wang, Rong Han, Yun-Fei Du, Yun-Fei Yao, Jun-Jie Chang, 2020. Impacts of mechanisms to promote participation in climate mitigation: border carbon adjustments versus uniform tariff measures. Climate Change Economics 11, 2041007.
- ↑ Li-Jing Liu, Felix Creutzig, Yun-Fei Yao, Yi-Ming Wei, Qiao-Mei Liang, 2020. Environmental and economic impacts of trade barriers: The example of China–US trade friction. Resource and Energy Economics 59, 101144.
- ↑ Kun Zhang, Qiao-Mei Liang, Li-Jing Liu, Mei-Mei Xue, Bi-Ying Yu, Ce Wang, Rong Han, Yun-Fei Du, Yun-Fei Yao, Jun-Jie Chang, 2020. Impacts of mechanisms to promote participation in climate mitigation: border carbon adjustments versus uniform tariff measures. Climate Change Economics 11, 2041007.
3.1) Production system and representation of economic sectors - C3IAM
C3IAM/GEEPA includes 27 sectors, which are paddy rice, wheat, cereal grains, vegetables and fruit and nuts, oil seeds, sugar cane and sugar beet, plant-based fibers, crops, cattle and sheep and goats and horses, animal products, raw milk, wool and silk-worm cocoons, forestry, fishing, coal, oil, gas, other minerals, other manufacturing, energy-intensive manufacturing, roil, electricity, gas manufacture and distribution, water, construction, transportation service industry and other services (shown in Table 1 below). C3IAM/MR.CEEPA covers 31 provinces and municipalities (without Hong Kong, Macao and Taiwan due to data availability) of China and includes 23 sectors (see Table 2 below).
GEEPA 27 sectors | GTAP 57 sectors | ||
Description in GTAP database 9 | |||
1 | pdr | pdr | Paddy rice |
2 | wht | wht | Wheat |
3 | gro | gro | Cereal grains, not elsewhere classified (n.e.c.) |
4 | v_f | v_f | Vegetables, fruit, nuts |
5 | osd | osd | Oil seeds |
6 | c_b | c_b | Sugar cane, sugar beet |
7 | pfb | pfb | Plant-based fibers |
8 | ocr | ocr | Crops n.e.c. |
9 | ctl | ctl | Cattle, sheep, goats, horses |
10 | petr | oap | Animal products n.e.c. |
11 | rmk | rmk | Raw milk |
12 | wol | wol | Wool, silk-worm cocoons |
13 | for | frs | Forestry |
14 | fsh | fsh | Fishing |
15 | col | Coal | Coal |
16 | oil | Oil | Oil |
17 | gas | Gas | Gas |
18 | omn | OtherMin | Minerals n.e.c. |
19 | cmt | OtherMnfc | Meat: cattle, sheep, goats, horse |
20 | omt | Meat products n.e.c. | |
21 | vol | Vegetable oils and fats | |
22 | mil | Dairy products | |
23 | pcr | Processed rice | |
24 | sgr | Sugar | |
25 | ofd | Food products n.e.c. | |
26 | b_t | Beverages and tobacco products | |
27 | tex | Textiles | |
28 | wap | Wearing apparel | |
29 | lea | Leather products | |
30 | lum | Wood products | |
31 | ppp | EintMnfc | Paper products, publishing |
32 | p_c | Roil | Petroleum, coal products |
33 | crp | EintMnfc | Chemical, rubber, plastic prods |
34 | nmm | Mineral products n.e.c. | |
35 | i_s | Ferrous metals | |
36 | nfm | Metals n.e.c. | |
37 | fmp | Metal products | |
38 | mvh | OtherMnfc | Motor vehicles and parts |
39 | otn | Transport equipment n.e.c. | |
40 | ele | Electronic equipment | |
41 | ome | Machinery and equipment n.e.c. | |
42 | omf | Manufactures n.e.c. | |
43 | ely | Elec | Electricity |
44 | gdt | FuelGas | Gas manufacture, distribution |
45 | wtr | Water | Water |
46 | cns | Cons | Construction |
47 | trd | OthServices | Trade |
48 | otp | TransService | Transport n.e.c. |
49 | wtp | Sea transport | |
50 | atp | Air transport | |
51 | cmn | OthServices | Communication |
52 | ofi | Financial services n.e.c. | |
53 | isr | Insurance | |
54 | obs | Business services n.e.c. | |
55 | ros | Recreation and other services | |
56 | osg | PubAdmin/Defence/Health/Educat | |
57 | dwe | Dwellings |
No. | Sectors | Sectoral Description |
1 | AGRI | Agriculture |
2 | Coal | Mining and Washing of Coal |
3 | Oil | Extraction of Petroleum |
4 | NatGAS | Extraction of Natural Gas |
5 | OtherMin | Mining of Other Ores |
6 | FoodTob | Manufacture of Foods and Tobacco |
7 | Textile | Manufacture of Textile |
8 | WearApp | Manufacture of Textile, Wearing Apparel and Accessories, Leather, Fur, Feather and Related Products and Footwear |
9 | WoodProd | Processing of Timber, Manufacture of Wood, Bamboo, Rattan, Palm, and Straw Products |
10 | PaperProd | Manufacture of Paper and Paper Products |
11 | Petr | Manufacture of refined petroleum products |
12 | Coking | Manufacture of coke |
13 | Chemistry | Manufacture of Raw Chemical Materials and Chemical Products |
14 | NonMetProd | Manufacture of Non-metallic Mineral Products |
15 | MetalSmelt | Smelting and Pressing of Ferrous Metals and Non-ferrous Metals |
16 | Metalware | Manufacture of Metal Products |
17 | Equipment | Manufacture of Machinery |
18 | ELEC | Production and Supply of Electricity and Heat |
19 | GasPandS | Production and Supply of Gas |
20 | WaterProSup | Production and Supply of Water |
21 | Construction | Construction |
22 | TraStorPost | Transport Service |
23 | OtherService | Other Service |
In the production module, we assume that each sector produce only one commodity, and the inputs of each sector include labor, capital, energy, and other intermediate inputs. In addition, the production of each sector is represented by a nested constant elasticity of substitute (CES) function, as shown in Equation (1). The CES function is characterized by the flexibility to reflect the alternatives between producers and consumers based on the price and cost. For example, if carbon pricing policies are implemented in the economy, the producers will choose to use lower-carbon energy for its production activities due to increases in the cost of high-carbon energy, thus promotes the economy transition from high-carbon energy to clean energy.
(1)
Where refer to the output of sector in region , is the input of sector in region , is shift parameter, is the share parameter, is the substitution parameter, is the elasticity of substitution.
In C3IAM, the substitution of production functions consists primarily of substitutions between electricity and non-ELEC bundle, energy aggregate and capital, as well as energy-capital aggregate and labor.
3.2) Capital and labour markets - C3IAM
3.3) Monetary instruments - C3IAM
3.4) Trade - C3IAM
Taking foreign trade into account, we adopts Armington assumption, which assumes there is imperfect substitutability between imports and domestic output sold domestically. The degree to which domestic and imported goods differ is reflected by the elasticity of substitution between them. Changes in the relative shares of foreign and domestic goods in the composite are determined by changes in the relative prices of these goods at home and abroad, given the Armington substitution elasticity and the initial shares of these goods in the benchmark SAM (Social Accounting Matrix). The commodity that supplied domestically is composed of domestic and imported commodities following a CES function. Furthermore, domestic commodity is used to meet domestic demands and for exports. In C3IAM, we use a constant elasticity transformation (CET) function to allocate total domestic output between exports and domestic sales, shown in Equations. (1) and (2).
(1)
(2)
Where andrespectively represent exports and domestic sales of domestically produced good in region ; and respectively represent export price and domestic sale price of domestically produced good in region ; and
respectively represent the shift parameter and share parameter in transformation function;
and respectively represent the substitution parameter and substitution elasticity in CET function between export and domestic sales.
3.5) Technological change - C3IAM
Technological change is an important source of economic growth. In C3IAM, in addition to the exogenous augmentation of the supplies of labor and natural resources, energy consumption per unit of output decreases externally over time (the so-called autonomous energy efficiency improvement index, AEEI), which is a heuristic representation of non-price-driven changes in energy use over time[1].
Technological change in electricity sector also has been specified with two advanced generation technologies: natural gas combined cycle technology with carbon capture and sequestration (NGCC-CCS) and integrated coal gasification technology with carbon capture and sequestration (IGCC-CCS). If these technologies are economically competitive with existing technologies, they will endogenously enter.
Technological change in agricultural sector plays an important role for meeting future demands of various agricultural products. The endogenous implementation of agricultural technological change is based on production costs and the impact of R&D investment on yield improvement which evolves with agricultural land use intensity. Land use intensity descripts the degree of yield amplification caused by human activities and the value in base year is estimated by empirical regress analysis. Investing technological changes not only enhance crop yield but only increase land use intensity, which raise costs for further yield increases in turn.
References
- ↑ Sergey Paltsev, John M Reilly, Henry D Jacoby, Richard S Eckaus, James R McFarland, Marcus C Sarofim, Malcolm O Asadoorian, Mustafa HM Babiker, 2005. The MIT emissions prediction and policy analysis (EPPA) model: version 4. MIT Joint Program on the Science and Policy of Global Change.
4) Energy - C3IAM
C3IAM includes detailed sectoral representation in energy sectors. In production activities, energy is an input in the nested CES production function. Total energy input is aggregated by electricity input and non-electricity energy input, and then there are substitutions between energy input and capital, as well as energy-capital aggregate and labor. In primary energy production activities (coal, crude oil and gas), the fossil energy resources are allowed to substitute with non-resource inputs in the top nest. The energy transformation sectors are represented by electricity sector with multi power generation technologies and refined oil sector. For household, energy substitutes with other goods or services following CD (Cobb-Douglas) function.
4.1) Energy resource endowments - C3IAM
The energy resource endowments include both fossil energy and non-fossil energy resources. Fossil resources comprise coal, oil, and gas. Non-fossil energy resources include nuclear, hydro, wind, solar, and other renewable resources.
4.1.1) Fossil energy resources - C3IAM
Fossil energy resources are subject to depletion based on physical production of related fuel in the previous period. Because C3IAM solves on a five-year time-step we approximate depletion in intervening years by multiplying the production of each fuel resource by a factor of five. As follows:
Where R represents fossil energy resource (including coal, oil, and gas) in region r, X represents the production of fossil energy in the previous period.
4.1.2) Uranium and other fissile resources - C3IAM
4.1.3) Bioenergy - C3IAM
4.1.4) Non-biomass renewables - C3IAM
C3IAM uses an additional production input called the ‘fixed factor’ to describes the representative capacity building constraint of energy technologies. The fixed factor is used to represent the specialized resources that are required for capacity building such as knowledgeable engineering, specialized manufacturing and services. The price of the fixed factor will therefore affect the rate at which this technology enters the market. If the demand for the technology is high, the fixed factor price representing the limited resources will also be high, thereby limiting the initial rate of expansion of production from new energy technologies and taking into consideration the adjustment costs [1].
The fixed factor also represents innovation in the form of learning-by-doing, which shows that the constraint is less binding as production and experience is gained. The representative agent is endowed with a very small amount of the particular fixed factor resource for each technology in the base year[2], andfor the base year is 0.00001 in the model. The amount of fixed factor then is increased as a function of cumulative production of that technology, representing cost reduction as we learn and gain experience. The equation for endowment is based on the forms in McFarland et al. (2004)[3], Jacoby et al. (2005) [2] and Ereira et al. (2010) [1]:
Where is the electricity output for a given technology in period t. is approximately linear with and . This term governs the growth of the fixed factor at low levels of output as. The term accelerates fixed factor growth at high levels of output as and[3].
References
- ↑ 1.0 1.1 Eleanor Charlotte Ereira, 2010. Assessing early investments in low carbon technologies under uncertainty: the case of Carbon Capture and Storage. Massachusetts Institute of Technology.
- ↑ 2.0 2.1 Henry D Jacoby, John M Reilly, James R McFarland, Sergey Paltsev, 2006. Technology and technical change in the MIT EPPA model. Energy Economics 28, 610-631.
- ↑ 3.0 3.1 J.R. McFarland, J.M. Reilly, H.J. Herzog, 2004. Representing energy technologies in top-down economic models using bottom-up information. Energy Economics. 26, 685–707.
4.2) Energy conversion - C3IAM
The core of energy system is to convert primary energy into secondary energy through specific energy conversion technology. Energy conversion occurs in production sectors and are represented by electricity sector with multi power generation technologies and refined oil sector.
While crude oil is retained in this nest structure for completeness, crude oil is not used directly in any other sector except in the refined oil sector. The refined oil sector uses crude oil as a “feedstock” to produce refined oil products. Crude oil thus enters not as part of the energy bundle but as a Leontief intermediate input. In the electricity sector, the production structure reflects the mutual substitution of 10 generation technologies, including traditional fossil energy generation technologies such as coal, oil and gas, as well as clean energy generation technologies such as wind energy, solar energy, and advanced technologies with carbon capture and sequestration (CCS) generation including NGCC (Natural Gas Combined Cycle)-CCS, IGCC (Integrated Gasification Combined Cycle)-CCS.
4.2.1) Electricity - C3IAM
The production structure for electricity sector is the most detailed among the sectors (Figure 1), which allows substitution of different generation technologies. These include generation technologies that exist in the base year data (conventional fossil energy, nuclear, and hydro) and advanced technologies that did not exist in the base year data.
Most of these advanced technologies have emerged as perfect substitutes for existing technologies. The only exception is wind and solar technology, which goes to the very top of the nest, and substitutes for other electricity technologies. Treating them as imperfect substitutes represents the uniqueness of these renewable technologies. While they can be well suited to some remote locations, they also suffer from intermittency that can increase their cost if they were to provide a large share of electricity production. Alternative substitute parameter allows gradual penetration only if the prices of other generation technologies continue to rise, and tends to limit the share of electricity that can be generated by wind and solar.
The lower nests in Figure 1 represent the structure within particular generation technologies. However, conventional fossil fuels do not separately represent coal, oil, and gas generation technologies, but instead deal with these technologies through direct substitution among the fuels.
Nuclear and hydro are with much simpler structures, concentrating on related resources, capital and labor. In both cases, resources are represented as fixed factor endowments that are specific to technology and region. Over time, changes in resources are externally controlled.
The two advanced fossil electricity generation technologies have similar structures. These technologies represent a natural gas combined cycle technology with carbon capture and sequestration (NGCC-CCS), and an integrated coal gasification technology with carbon capture and sequestration (IGCC-CCS). The elaborated production structures for these technologies also include energy and capital penalty for capture and sequestration.
4.2.2) Heat - C3IAM
4.2.3) Gaseous fuels - C3IAM
4.2.4) Liquid fuels - C3IAM
4.2.5) Solid fuels - C3IAM
4.2.6) Grid, pipelines and other infrastructure - C3IAM
4.3) Energy end-use - C3IAM
C3IAM defines the transport, industry and residential/commercial as end-use sectors. For these sectors, the model can effectively simulate the possible technological progress, efficiency improvement, cost reduction and technological breakthrough in the development of the medium and long term. In combination with current policies and future emission-reduction targets in these sector, the energy consumption under different scenarios will be analyzed.
More details on the transport, industry and residential modelling can be found on the transport, industrial sector, and residential and commercial sectors pages.
4.3.1) Transport - C3IAM
C3IAM/NET-Transport model divides the transport sector into three parts, including intercity passenger transport, urban passenger transport and freight transport. Urban passenger transport is divided into public transport (bus and rail transit), taxi, and private vehicles (cars and electric bikes). Intercity passenger transport is divided into private vehicles and four main business intercity passenger transport (road transport, railway transport, aviation and waterway transport). Freight transport is divided into five main types: road transport, railway transport, aviation transport, waterway and pipeline transport. The main fuel includes gasoline, diesel, LNG, electricity, jet fuel, biofuel, fuel oil, hydrogen and so on.
By considering the economic development, the trend of population growth, the development of service industry and the level of transportation infrastructure in the future, combined with the change of travel behavior and the popularization of shared mobility, the multi-factor regression method is used to predict the intercity passenger travel demand [1]. By considering the increase of urbanization and per capita income, the urban passenger travel demand is predicted [2]. By considering the future growth rate of GDP, the change of industrial structure, the development of e-commerce and other factors, the freight transport demand is predicted.
With the goal of minimizing the annualized cost during the planning period, the model can directly describe the competition and substitution process of different technologies in the transport sector and describe the evolution of the transport structure. The model can effectively simulate the possible technological progress, efficiency improvement, cost reduction and technological breakthrough in the development of the medium and long term transport sector. In combination with current transport policies and future emission-reduction targets in the transportation sector, the energy consumption and CO2 emission-reduction potential under different scenarios will be analyzed. A detailed and operable development path for China’s transport sector can then be provided.
References
4.3.2) Residential and commercial sectors - C3IAM
In C3IAM/NET, the residential and commercial sectors part can be used to simulate the energy flow of building equipment (such as coal stove, air conditioner, water heater, etc.) using various kinds of energy (coal, oil, natural gas, electricity, solar energy, biomass and geothermal energy) to meet the service demand (space heating, cooling, hot water, cooking, lighting and others), so as to calculate the energy consumption and GHG emissions of various equipment combinations. The technology pathway with the optimal cost can be obtained under the constraints of specific realistic conditions, and meet the energy service demand of buildings at the same time. Energy service demand prediction module, energy - environment - technology - policy evaluation module and output module make up the residential and commercial sectors of C3IAM.
Based on socioeconomic variables, climate conditions and related policy planning, the energy service demand prediction module determines the future demand of the residential and commercial sectors for space heating, cooling, hot water, cooking, lighting and other energy services.
Energy - environment - technology - policy evaluation module aims to minimize the total cost, on the basis of multifaceted data such as energy service demand from the energy service demand prediction module, investment costs, operation and maintenance costs, energy efficiencies, energy prices and carbon emission factors. Technical progress is represented as efficiency improvements and cost reductions here. The constraints include carbon emission, energy supply, policy restriction and technical level.
Output module shows the total energy consumption and GHG emissions of the residential and commercial sector from 2020 to 2060. Indirect emissions from electricity are also taken into account. The energy structure and emission sources can also be distinguished and analyzed. Besides, the output module provides the development pathway of each kind of device.
4.3.3) Industrial sector - C3IAM
C3IAM/NET-industrial sectors include steel, cement, nonferrous metals, chemical, paper and other industries. First, on the basis of considering economic development, industrial upgrading, acceleration of urbanization, intelligentization, electrification and other social and economic changes, the demand for products of each industrial sector is forecasted separately. Secondly, by incorporating factors such as technological progress, raw material substitution, fuel substitution, process adjustment, etc., the production process of each industrial sector is simulated to obtain the corresponding energy flow and material flow under the optimal production cost.
The path optimization part in industrial sectors is based on the technical perspective, considering more than 200 energy-saving technologies (such as non-blast furnace steelmaking, hydrogen steelmaking, new dry kiln, waste heat power generation technology, biological conversion technology, electrolytic water hydrogen production, etc.). By setting a series of technology, energy and emission parameters such as technology investment cost, energy conversion efficiency, energy emission factor, etc., the industrial sectors are modeled. With the goal of minimizing the total annual cost, the model chooses the optimal technological development path for the industrial sector of each region or the country under multiple constraints such as backward production capacity phase-out, technology substitution, fuel conversion, and technological progress. The model of the industrial sectors reflects the characteristics of the industrial production process with a wide variety of products, production processes, and diverse energy-saving technologies. The biggest advantage lies in the bottom-up view of actual production, making the simulation process and results practical. The results show the future potential of electric arc furnaces in the iron and steel industry [1], the emission reduction potential of raw material substitution in the cement industry [2], and the emission reduction path of multiple products in the chemical industry [3], which can provide the government and enterprises with detailed and feasible technical investment guidance.
References
- ↑ Runying An, Biying Yu, Ru Li, Yi-Ming Wei, 2018. Potential of energy savings and CO2 emission reduction in China’s iron and steel industry. Applied energy 226, 862-880.
- ↑ Cheng-Yao Zhang, Biying Yu, Jing-Ming Chen, Yi-Ming Wei, 2021. Green transition pathways for cement industry in China. Resources, Conservation and Recycling 166, 105355.
- ↑ Jing-Ming Chen, Biying Yu, Yi-Ming Wei, 2018. Energy technology roadmap for ethylene industry in China. Applied Energy 224, 160-174.
4.3.4) Other end-use - C3IAM
4.4) Energy demand - C3IAM
In C3IAM, the energy demand is part of the production functions for each sector. For each sector’s production, different inputs including labor, capital and energy can be substituted for each other. Energy use efficiency could improve through time exogenously due to technical change or economic structural change.
The energy demand is primarily driven by economic growth, which is calibrated based on the growth rate of total factor productivity.
4.5) Technological change in energy - C3IAM
In C3IAM, technological change in energy is reflected by energy consumption per unit of output decreases externally over time (the so-called autonomous energy efficiency improvement index, AEEI), which has an energy use efficiency improvement over time.
Technological change in electricity sector also has been specified with two advanced generation technologies: natural gas combined cycle technology with carbon capture and sequestration (NGCC-CCS) and integrated coal gasification technology with carbon capture and sequestration (IGCC-CCS). If these technologies are economically competitive with existing technologies, they will endogenously enter.
5) Land-use - C3IAM
The future patterns of land use have direct influence on GHG emissions and mitigation potential for land use sector and food supply. The C3IAM/EcoLa model is a global multi-regional land use allocation optimization model, which covers the agricultural and forestry sectors (see the following Figure 1). It can be used to analyze land use change in a long-term period. The primary objective of the model is to minimize the total cost of production under consideration of agricultural demand in 12 regions.
Major types of cost in C3IAM/EcoLa are:
(1) Production costs of crop and livestock production, which are obtained by a total sum of the costs of labor, capital and intermediate inputs divided by the land area obtained from C3IAM/GEEPA;
(2) Land conversion costs which are exogenously determined by the cost of new additional land and investment into infrastructure;
(3) Carbon emissions costs which consider the carbon costs caused by land use change in mitigation scenarios.
5.1) Agriculture - C3IAM
Primary agricultural products considered in the model are listed in the following Table 1. The livestock activities are connected with the feed requirement per animal product. Following Alcamo’s work [1], the model currently considers ruminants for livestock activities such as cattle and sheep, but non-ruminants are not included. The total forage demand is calculated by multiplying livestock unit with average forage consumption per livestock unit during one year.
For the reference land use area distribution used in the base year 2011, croplands are produced by eight crop categories which contain 149 crop types (see Table 1). According to Food and Agriculture Organization (FAO) definition, grass is from permanent pastures and can be used to graze [2].
Crop types | Concrete products |
Rice | rice |
Wheat | wheat |
CerealCrop | barley, buckwheat, canary seed, cereals, maize, millet, mixed grain, quinoa, rye, sorghum, triticale |
VegCrop | almonds, apples, arecanuts, avocados, bambara beans, bananas, beans, berries, blueberries, brazil nuts, broad beans, horse beans, cabbages and other brassicas, carrots and turnips, cashew nuts, cashewapple, cassava, cauliflowers and broccoli, cherries, chestnuts, chick peas, chicory roots, chillies and peppers, citrus fruit, coconuts, cow peas, cranberries, cucumbers and gherkins, currants, dates, eggplants, figs, tropical fruit, garlic, gooseberries, grapefruit, grapes, hazelnuts, kiwi fruit, leeks, leguminous vegetables, lemons and limes, lentils, lettuce and chicory, lupins, mangoes, mushrooms and truffles, nuts, oats, okra, olives, onions, oranges, other melons, papayas, peaches and nectarines, pears, persimmons, pigeon peas, pineapples, pistachios, plantains, plums and sloes, pome fruit, potatoes, pulses, pumpkins, quinces, raspberries, roots and tubers, spinach, stone fruit, strawberries, string beans, sweet potatoes, tangerines, mandarins, taro, tomatoes, walnuts, watermelons, yams, yautia |
OilCrop | castor oil seed, groundnuts, hempseed, jojoba seeds, kapokseed, karate nuts, linseed, melonseed, mustard seed, oilpalm, oilseeds, poppy seed, rapeseed, safflower seed, sesame, soybeans, sunflower, tallowtree Seeds, tung nuts |
SugarCrop | sugar beet, sugar beet |
FiberCrop | agave, fibrenes, hemp tow waste, jute, manila fibre, other bastfibres, ramie, sisal |
OtherCrop | anise, apricots, artichokes, asparagus, carobs, cinnamon, cloves, cocoa, coffee, fonio, ginger, hops, kola nuts, maté, nutmeg, pepper, peppermint, pyrethrum, spices, tea, tobacco, vanilla, vetches |
Livestock | cattle, goats, horses, sheep |
References
- ↑ Rüdiger Schaldach, Joseph Alcamo, Jennifer Koch, Christina Kölking, David M Lapola, Jan Schüngel, Jörg A Priess, 2011. An integrated approach to modelling land-use change on continental and global scales. Environmental Modelling & Software 26, 1041-1051.
- ↑ François Souty, Thierry Brunelle, Patrice Dumas, Bruno Dorin, Philippe Ciais, Renaud Crassous, Chistoph Müller, Alberte Bondeau, 2012. The Nexus Land-Use model version 1.0, an approach articulating biophysical potentials and economic dynamics to model competition for land-use. Geoscientific Model Development 5, 1297-1322.
5.2) Forestry - C3IAM
Forest sector is divided into managed forests and no-managed forests. The primary forest products are supplied from managed forests [1]. The built-up, water and ice areas are assumed constant during the study period.
References
- ↑ Petr Havlík, Hugo Valin, Mario Herrero, Michael Obersteiner, Erwin Schmid, Mariana C Rufino, Aline Mosnier, Philip K Thornton, Hannes Böttcher, Richard T Conant, 2014. Climate change mitigation through livestock system transitions. proceedings of the National Academy of Sciences 111, 3709-3714.
5.3) Land-use change - C3IAM
For the projection of land use change, C3IAM/EcoLa works on a time step of 5 years in a dynamic recursive mode. Future demand for regional agricultural and forest products (e.g., rice, wheat, cereals, vegetables, oil seeds, sugar, fibers, other crops, livestock and forestry) is exogenous, it relies on income per capita, and population projection of different regions based on GTAP database. Moreover, technical change for agricultural sector depends on different biophysical and socioeconomic factors [1][2]. Changes of agricultural productivity and crop productivity among 12 regions are different, what is more, SSP1-3 have different product specific rates. Trade in food and forest products across the various regions is not considered in the study.
=== References ===
5.4) Bioenergy land-use - C3IAM
5.5) Other land-use - C3IAM
5.6) Agricultural demand - C3IAM
5.7) Technological change in land-use - C3IAM
6) Emissions - C3IAM
In C3IAM model, we consider both GHG emissions and traditional air pollutant emissions. Besides energy-related carbon dioxide (CO2), CO2 from other sources, methane (CH4) and nitrous oxide (N2O) are treated as GHGs in the model. The traditional air pollutants considered are carbon monoxide (CO), sulfur dioxide (SO2), nitrous oxides (NOx), ammonia (NH3), black carbon (BC), organic carbon (OC) and non-methane volatile organic compounds (NMVOCs).
In the sub-sections of this chapter, the GHG and non-GHG emissions included in C3IAM are presented.
6.1) GHGs - C3IAM
C3IAM simulates long-lived GHG emissions of carbon dioxide (CO2), methane (CH4), and nitrous oxide (N2O). CO2 emissions from fuel combustion are calculated based on energy sources with fixed coefficient. CO2 resulting from land-use changes is endogenously calculated as a consequence of the land use (taking changes of land use from previous year). CO2 emissions from industrial process, CH4 and N2O emissions are basically associated with each sector’s activity level. The activity level and corresponding emissions in the base year are drawn from the Greenhouse Gas and Air Pollution Interactions and Synergies (GAINS)[3].
=== References ===
6.2) Pollutants and non-GHG forcing agents - C3IAM
C3IAM calculates emissions of aerosols and ozone precursors (SO2, NOx, PM2.5, BC, OC, CO, NMVOCs, and NH3). SO2 emission is a major source of aerosols that are thought to have a cooling effect, and other gases are all important for the climate and chemistry components.
These air pollutants emissions in the base year are calibrated to the GAINS (Greenhouse gas–Air pollution Interactions and Synergies) model. The GAINS emission scenarios are used as input to C3IAM frameworks to characterize the long-term air pollution trajectories.
6.3) Carbon dioxide removal (CDR) options - C3IAM
The CDR options modelled in C3IAM include CCS and BECCS in power generation, as described in Micro-economy section.
The CCS technology is used in power plants with coal and gas, and the BECCS technology is used in power plants with bioenergy.
There is no direct air capture option in C3IAM.
7) Climate - C3IAM
C3IAM includes an internal climate module -- C3IAM/Climate, which translates the regional emissions into global temperature and radiative forcing through atmospheric concentrations. Alternatively, C3IAM allows a soft link with the MAGICC6 climate model for reporting a number of climate outcomes based on this widely used model. Besides, C3IAM includes the Climate Change Loss Model (C3IAM/Loss), which can assess the impacts of temperature/sea level/extreme events [4].
References
7.1) Modelling of climate indicators - C3IAM
The C3IAM/Climate module represents the climate component, and the emission information generated from C3IAM/GEEPA is fed into C3IAM/Climate. We used C3IAM/Climate to calculate climate indicators such as global mean temperature changes and radiative forcing. Here the Climate module is developed according to the Beijing Climate Center Climate System Model (BCC_CSM), which is one of the earth system models that participated in CMIP5 simulations for the IPCC AR5 (see Figure 1 for the framework). The BCC_CSM has four component models, i.e., Global Atmosphere Model (BCC_AGCM2.1), Land Surface Model (BCC_AVIM1.0), Global Ocean Model (MOM4_L40v1) and Global Thermodynamic Sea Ice Model (SIS). These component models are inter-related and interacted with each other through fluxes of energy, momentum and water. The flux coupler was based on that of NCAR/CCSM2. The BCC_CSM is a fully coupled Climate–Carbon Cycle Model, including oceanic and terrestrial carbon cycle with dynamical vegetation. The atmospheric CO2 concentration and its temporal evolution can be well reproduced when forced by anthropogenic emissions of CO2. In addition to the long-term climate change simulations and projections, BCC_CSM has also been used for short-term climate predictions, as well as the Sub-seasonal to Seasonal (S2S) Prediction Project.
7.2) Climate damages, temperature changes - C3IAM
The damages are considered at the macro and sectoral levels. In the C3IAM/EcOp, the relationship between temperature variation and total economic output is incorporated as the damage function. In the C3IAM/GEEPA&MR.CEEPA, the climate impacts on agriculture, labor productivity, energy, health, extreme events, and so on are included. The damages are calculated with the climate variables at the grid level.
8) Non-climate sustainability dimension - C3IAM
Non-climate sustainability is not currently modelled in C3IAM.
8.1) Air pollution and health - C3IAM
8.2) Water - C3IAM
8.3) Other materials - C3IAM
8.4) Other sustainability dimensions - C3IAM
9) Appendices - C3IAM
9.1) Mathematical model description - C3IAM
9.2) Data - C3IAM
Global Trade Analysis Project (GTAP):https://www.gtap.agecon.purdue.edu
Greenhouse Gas - Air Pollution Interactions and Synergies(GAINS): https://gains.iiasa.ac.at/models/gains_models3.html
Coupled Model Intercomparison Project Phase 5(CMIP5): https://esgf-node.llnl.gov/projects/cmip5
Center for Sustainability and the Global Environment (SAGE): http://nelson.wisc.edu/sage/data-and-models/datasets.php
Land-Use Harmonization (LUH2): https://luh.umd.edu/data.shtml
UNEP-WCMC: http://sea.unep-wcmc.org/wdbpa/
SSP Database (Shared Socioeconomic Pathways) : https://tntcat.iiasa.ac.at/SspDb/dsd?Action=htmlpage&page=10
10) References - C3IAM
[1] Yi-Ming Wei, Rong Han, Qiao-Mei Liang, Bi-Ying Yu, Yun-Fei Yao, Mei-Mei Xue, Kun Zhang, Li-Jing Liu, Juan Peng, Pu Yang, Zhi-Fu Mi, Yun-Fei Du, Ce Wang, Jun-Jie Chang, Qian-Ru Yang, Zili Yang, Xueli Shi, Wei Xie, Changyi Liu, Zhongyu Ma, Jinxiao Tan, Weizheng Wang, Bao-Jun Tang, Yun-Fei Cao, Mingquan Wang, Jin-Wei Wang, Jia-Ning Kang, Ke Wang, Hua Liao, 2018. An integrated assessment of INDCs under Shared Socioeconomic Pathways: an implementation of C3IAM. Natural Hazards 92, 585-618.
[2] Brian C O’Neill, Elmar Kriegler, Keywan Riahi, Kristie L Ebi, Stephane Hallegatte, Timothy R Carter, Ritu Mathur, Detlef P van Vuuren, 2014. A new scenario framework for climate change research: the concept of shared socioeconomic pathways. Climatic change 122, 387-400.
[3] Yi-Ming Wei, Rong Han, Ce Wang, Biying Yu, Qiao-Mei Liang, Xiao-Chen Yuan, Junjie Chang, Qingyu Zhao, Hua Liao, Baojun Tang, Jinyue Yan, Lijing Cheng, Zili Yang, 2020. Self-preservation strategy for approaching global warming targets in the post-Paris Agreement era. Nature Communications 11, 1624.
[4] Kun Zhang, Qiao-Mei Liang, Li-Jing Liu, Mei-Mei Xue, Bi-Ying Yu, Ce Wang, Rong Han, Yun-Fei Du, Yun-Fei Yao, Jun-Jie Chang, 2020. Impacts of mechanisms to promote participation in climate mitigation: border carbon adjustments versus uniform tariff measures. Climate Change Economics 11, 2041007.
[5] Li-Jing Liu, Felix Creutzig, Yun-Fei Yao, Yi-Ming Wei, Qiao-Mei Liang, 2020. Environmental and economic impacts of trade barriers: The example of China–US trade friction. Resource and Energy Economics 59, 101144.
[6] Sergey Paltsev, John M Reilly, Henry D Jacoby, Richard S Eckaus, James R McFarland, Marcus C Sarofim, Malcolm O Asadoorian, Mustafa HM Babiker, 2005. The MIT emissions prediction and policy analysis (EPPA) model: version 4. MIT Joint Program on the Science and Policy of Global Change.
[7] Eleanor Charlotte Ereira, 2010. Assessing early investments in low carbon technologies under uncertainty: the case of Carbon Capture and Storage. Massachusetts Institute of Technology.
[8] Henry D Jacoby, John M Reilly, James R McFarland, Sergey Paltsev, 2006. Technology and technical change in the MIT EPPA model. Energy Economics 28, 610-631.
[9] Bao-Jun Tang, Xiao-Yi Li, Biying Yu, Yi-Ming Wei, 2019. Sustainable development pathway for intercity passenger transport: A case study of China. Applied Energy 254, 113632.
[10] Xi Li, Biying Yu, 2019. Peaking CO2 emissions for China's urban passenger transport sector. Energy Policy 133, 110913.
[11] Runying An, Biying Yu, Ru Li, Yi-Ming Wei, 2018. Potential of energy savings and CO2 emission reduction in China’s iron and steel industry. Applied energy 226, 862-880.
[12] Cheng-Yao Zhang, Biying Yu, Jing-Ming Chen, Yi-Ming Wei, 2021. Green transition pathways for cement industry in China. Resources, Conservation and Recycling 166, 105355.
[13] Jing-Ming Chen, Biying Yu, Yi-Ming Wei, 2018. Energy technology roadmap for ethylene industry in China. Applied Energy 224, 160-174.
[14] Rüdiger Schaldach, Joseph Alcamo, Jennifer Koch, Christina Kölking, David M Lapola, Jan Schüngel, Jörg A Priess, 2011. An integrated approach to modelling land-use change on continental and global scales. Environmental Modelling & Software 26, 1041-1051.
[15] François Souty, Thierry Brunelle, Patrice Dumas, Bruno Dorin, Philippe Ciais, Renaud Crassous, Chistoph Müller, Alberte Bondeau, 2012. The Nexus Land-Use model version 1.0, an approach articulating biophysical potentials and economic dynamics to model competition for land-use. Geoscientific Model Development 5, 1297-1322.
[16] Petr Havlík, Hugo Valin, Mario Herrero, Michael Obersteiner, Erwin Schmid, Mariana C Rufino, Aline Mosnier, Philip K Thornton, Hannes Böttcher, Richard T Conant, 2014. Climate change mitigation through livestock system transitions. proceedings of the National Academy of Sciences 111, 3709-3714.
[17] Thanh Binh Nguyen, Fabian Wagner, Wolfgang Schoepp, 2011. GAINS–an interactive tool for assessing international GHG mitigation regimes, International Conference on Information and Communication on Technology. Springer, pp. 124-135.
[18] Xiao-Chen Yuan, Nan Zhang, Wei-Zheng Wang, Yi-Ming Wei, 2021. Large-scale emulation of spatio-temporal variation in temperature under climate change. Environmental Research Letters 16, 014041.
- ↑ F Ewert, MDA Rounsevell, I Reginster, MJ Metzger, R Leemans, 2005. Future scenarios of European agricultural land use: I. Estimating changes in crop productivity. Agriculture, Ecosystems & Environment 107, 101-116.
- ↑ Stefan Wirsenius, Christian Azar, Göran Berndes, 2010. How much land is needed for global food production under scenarios of dietary changes and livestock productivity increases in 2030? Agricultural systems 103, 621-638.
- ↑ Thanh Binh Nguyen, Fabian Wagner, Wolfgang Schoepp, 2011. GAINS–an interactive tool for assessing international GHG mitigation regimes, International Conference on Information and Communication on Technology. Springer, pp. 124-135.
- ↑ Xiao-Chen Yuan, Nan Zhang, Wei-Zheng Wang, Yi-Ming Wei, 2021. Large-scale emulation of spatio-temporal variation in temperature under climate change. Environmental Research Letters 16, 014041.